How quantum computers work / Come funzionano i computer quantistici
How quantum computers work / Come funzionano i computer quantistici
Segnalato dal Dott. Giuseppe Cotellessa / Reported by Dr. Giuseppe Cotellessa
Quantum computing promises immense computational power beyond the fastest supercomputer available today.
Scientists hope to harness this power to solve problems that are difficult for traditional digital computers, like simulating molecules and proteins to create new materials and drugs, or crunching big data sets to supercharge artificial intelligence.
A variety of approaches exist to implement quantum computing, from trapping ions with lasers to confining electrons with semiconductor quantum dots. One tech company, for example, focuses on building quantum processors with superconducting circuits.
A common expectation is that quantum computers are mysterious machines that are locked away in high-tech research labs. For the most part, that is true.
But anyone in the world can try their hand at programming and carrying out experiments on real quantum computers located in IBM’s Quantum Computing Labs. The IBM Q Experience enables public access to these computers over the internet.
A quantum score composed on IBM’s Quantum Composer as part of the IBM Q Experience. Also shown are the results (as the quantum state computation basis) of the experiment, which was run on IBM’s Q 5 Tenerife quantum computer. / Un punteggio quantico composto su Quantum Composer di IBM come parte di IBM Q Experience. Vengono anche mostrati i risultati (come base di calcolo dello stato quantico) dell'esperimento, che è stato eseguito sul computer quantico IBM Q 5 di Tenerife. Source: IBM.
Quantum score
The journey begins by creating a set of instructions that will tell the quantum computer which computations to perform. This is done by designing a quantum algorithm using a web-based graphical user interface called the Quantum Composer.
Much like writing lines of musical notation to compose a song, this tool allows the user to drag and drop symbols on a chart to create what is known as a Quantum Score. The symbols represent quantum logic gates. In essence, the Quantum Score represents a quantum circuit that instructs the quantum computer to perform various operations. It is similar to how logic gates control a traditional digital computer.
Once the Quantum Score is complete, the program is sent to the cloud. There it is queued for processing on one of IBM’s quantum computers. Inside these computers are quantum processors comprised of several quantum bits, or “qubits.” These qubits, analogous to bits from classical computing, execute the quantum algorithm. But to carry out the proper instructions, the qubits must receive control signals in the appropriate format – in this case, microwave pulses.
The operations in the Quantum Score are sent to a stack of microwave electronics. There they are converted into microwave pulses of corresponding frequencies, phases and durations. These pulses are carried over coaxial cables to the quantum processor to manipulate the quantum states of the qubits on the chip to execute the program.
Dilution refrigerator
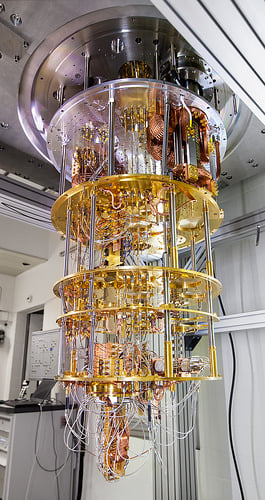
Each descending level of a dilution refrigerator is cooled to a lower temperature. The quantum chip rests at the bottom at a frigid 15 mK above absolute zero. Source: IBM
The processor itself is a tiny chip embedded in a circuit board only a few inches wide which rests at the bottom of a large dilution refrigerator. The chip is mounted within a cryoperm shield (a special magnetic shielding that is most effective at cryogenic temperatures) at the refrigerator's coldest level. This location is kept at temperatures below 15 milliKelvins – just above absolute zero and colder than the vacuum of space.
The dilution refrigerator can achieve such low temperatures by exploiting the endothermic heat of mixing between two isotopes of helium: helium-3 (3He) and helium-4 (4He). This is possible because the 3He-4He mixture experiences a spontaneous phase separation below 870 mK that results in a concentrated phase of nearly 100% 3He and a dilute phase consisting of around 6.6% 3He and 93.4% 4He. When the two phases enter the mixing chamber at the bottom of the refrigerator, a dilution process occurs as 3He travels from the concentrated phase to the dilute phase. Heat is extracted in the process.
This contraption is necessary because the quantum states of the qubits in the processor are extremely delicate. The slightest external disturbance--including heat, vibration and electromagnetic radiation--will destroy the quantum states in a process known as decoherence.
Meanwhile, the microwaves that carry control and measurement signals to the quantum chip are transmitted through coaxial cables. As the pulses – oscillating at frequencies around 4 GHz to 6 GHz – travel through each of the refrigerator’s stages, they pass through attenuators to ensure thermal noise does not reach the processor.
Quantum processor
An image of IBM’s five-qubit processor with its main elements highlighted, including the five qubits and their control, read-out and coupling resonators. / Un'immagine del processore IBM a cinque qubit con i suoi elementi principali evidenziati, inclusi i cinque qubit ei loro risuonatori di controllo, lettura e accoppiamento.Source: IBM Research.
IBM’s quantum chips consist of a silicon wafer that contains qubits and resonators composed of aluminum and niobium. The qubits are made in a pattern generated with electron-beam lithography. A resonator is connected to each qubit to deliver the control pulses that govern the qubit’s state. The qubits are made by ion etching a sputtered niobium film. Additional resonators link multiple qubits to enable entanglement.
An entangled qubit’s state cannot be described independently of its paired qubit. If the state of the first qubit is measured, the result of measuring the state of the second qubit is correlated to that of the first. Useful quantum computations often involve executing conditional logic operations in which one qubit’s state depends on that of another qubit.
Entanglement is one of the key quantum mechanical phenomena that provides the potential for immense computational acceleration among quantum computers compared to classical computers. Another key is superposition, which allows qubits to exist in multiple states at once. The value of a qubit is expressed as |0⟩ or |1⟩, analogous to a classical bit’s 0 or 1. The difference is that instead of existing as only 0 or 1, qubits can exist in a superposition of the two values.
A Bloch sphere can be used to visualize the superpositional state of a qubit, indicated as a vector with a certain angle θ from the z-axis and φ from the x-axis. Measuring the qubit’s state collapses its value to 0 or 1. / Una sfera di Bloch può essere usata per visualizzare lo stato di sovrapposizione di un qubit, indicato come un vettore con un certo angolo θ dall'asse z e φ dall'asse x. Misurare lo stato del qubit collassa il suo valore a 0 o 1.Source: IBM Research.
This superposition can be represented geometrically as a Bloch sphere. The north pole of the sphere represents the |0⟩ state, while the south pole represents the |1⟩ state. A superposition of the two states is represented by a vector pointing from the center of the sphere to somewhere on the surface between the two poles.
Only by measuring the qubit’s state does its value collapse to 0 or 1. The solution is like a probability distribution: the qubit will read out as 0 a certain percentage of the time and as 1 a different percentage of the time.
This behavior is represented by the formula |ψ⟩ = α|0⟩ + β|1⟩ with |α|2 + |β|2 = 1. When measured, the probability the qubit will collapse to 0 is |α|2and the probability the qubit will collapse to 1 is |β|2. The ability to exist in entangled states and in superpositions of states allows qubits to evaluate multiple solutions simultaneously.
IBM’s quantum processors use fixed-frequency, superconducting, Josephson-junction-based transmon qubits. A qubit of this type is essentially an LC resonator – a capacitor and an inductor connected in series. The inductor is the Josephson junction – a pair of superconducting electrodes that are separated by a very thin insulator that is a thousandth of a hair thick. The Josephson junction creates the nonlinearity that enables the LC resonator to act like an atom with two quantum energy levels: |0⟩ and |1⟩. Microwave control pulses manipulate the qubit between these two states to carry out quantum operations.
A schematic close-up of IBM’s superconducting qubit, including a zoomed-in view of its Josephson junction. / Un primo piano schematico del qubit superconduttore di IBM, inclusa una vista ingrandita della giunzione Josephson. Source: IBM Research.
To determine the result of a qubit’s operation, a measurement signal is sent as a microwave pulse to the qubit’s resonator. The measurement destroys the qubit’s superposition and collapses the qubit’s state to 0 or 1. The reflected signal travels back up the dilution refrigerator, passing through isolators that protect the qubits from noise as well as amplifiers that boost the signal’s strength enough to read the qubit’s state accurately. The signal then passes back through the stack of microwave electronics, this time to be converted from microwave frequency to a digital signal that is sent over the internet to the local computer to display the result of the quantum calculation.
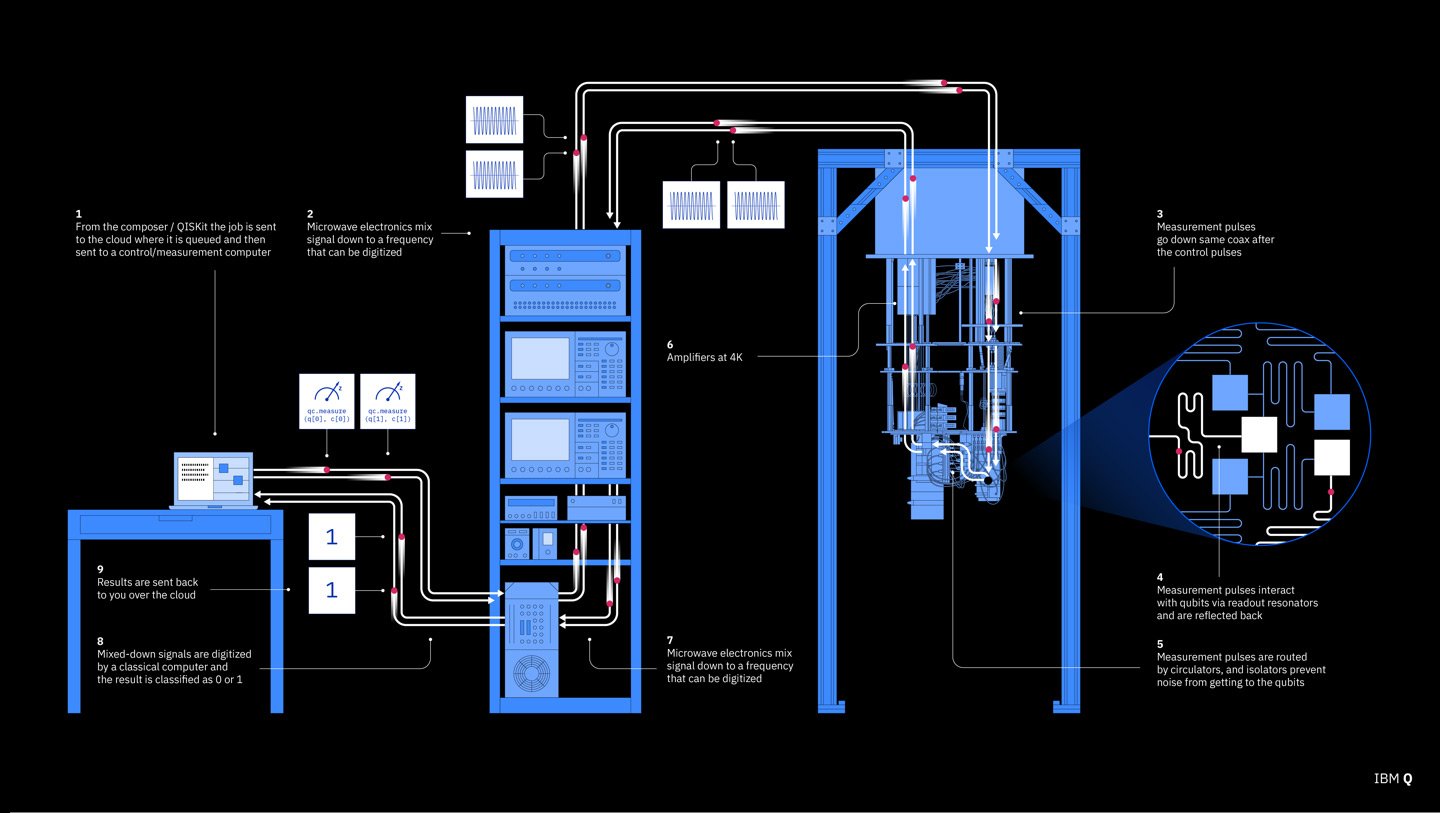
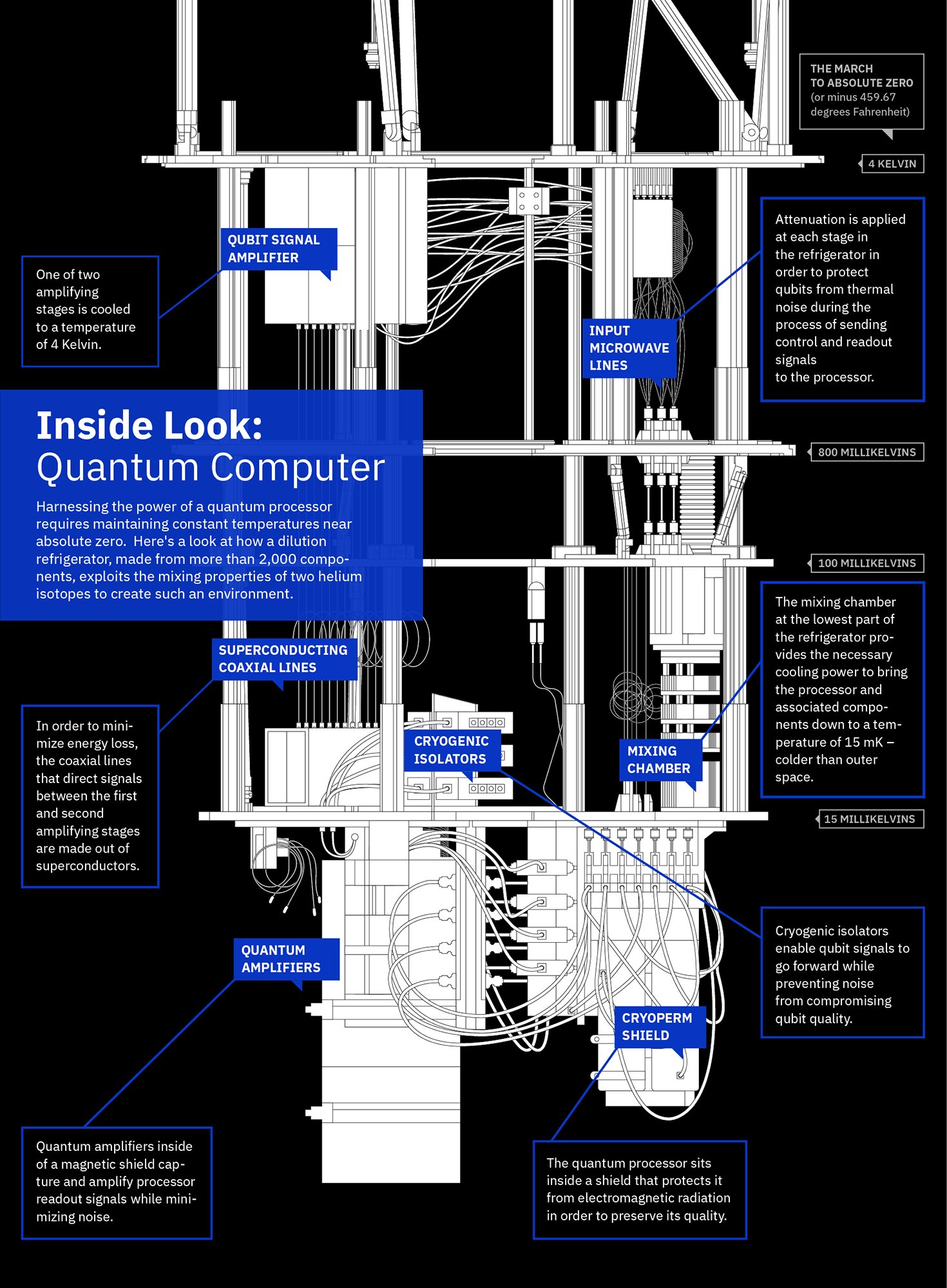
ITALIANO
Il calcolo quantico promette un'immensa potenza computazionale al di là del supercomputer più veloce disponibile oggi.
Gli scienziati sperano di sfruttare questo potere per risolvere i problemi che sono difficili per i computer digitali tradizionali, come simulare molecole e proteine per creare nuovi materiali e farmaci, o scricchiolare grandi set di dati per potenziare l'intelligenza artificiale.
Esistono diversi approcci per implementare il calcolo quantistico, dall'intrappolare gli ioni con i laser agli elettroni di confinamento con i punti quantici dei semiconduttori. Una società tecnologica, ad esempio, si concentra sulla costruzione di processori quantistici con circuiti superconduttori.
Un'aspettativa comune è che i computer quantistici sono macchine misteriose che sono rinchiuse in laboratori di ricerca high-tech. Per la maggior parte, è vero.
Ma chiunque nel mondo può cimentarsi nella programmazione e nell'esecuzione di esperimenti su computer quantistici reali situati nei Quantum Computing Labs di IBM. IBM Q Experience consente l'accesso pubblico a questi computer su Internet.
Punteggio quantico
Il viaggio inizia creando una serie di istruzioni che diranno al computer quantico quali calcoli eseguire. Questo viene fatto progettando un algoritmo quantistico utilizzando un'interfaccia utente grafica basata sul web chiamata Quantum Composer.
Proprio come scrivere linee di notazione musicale per comporre una canzone, questo strumento consente all'utente di trascinare e rilasciare simboli su un grafico per creare ciò che è noto come un punteggio quantico. I simboli rappresentano porte logiche quantistiche. In sostanza, il punteggio quantico rappresenta un circuito quantistico che istruisce il computer quantico a eseguire varie operazioni. È simile a come le porte logiche controllano un computer digitale tradizionale.
Una volta completato il punteggio quantico, il programma viene inviato al cloud. Qui viene accodato per l'elaborazione su uno dei computer quantistici di IBM. All'interno di questi computer ci sono processori quantici composti da diversi bit quantici, o "qubit". Questi qubit, analoghi ai bit del calcolo classico, eseguono l'algoritmo quantistico. Ma per eseguire le istruzioni corrette, i qubit devono ricevere segnali di controllo nel formato appropriato - in questo caso, impulsi a microonde.
Le operazioni nel punteggio quantico vengono inviate a una pila di componenti elettronici a microonde. Lì vengono convertiti in impulsi a microonde di frequenze, fasi e durate corrispondenti. Questi impulsi sono trasferiti su cavi coassiali al processore quantico per manipolare gli stati quantistici dei qubit sul chip per eseguire il programma.
Frigorifero per diluizione
Ogni livello discendente di un frigorifero di diluizione viene raffreddato a una temperatura inferiore. Il chip quantistico poggia sul fondo ad un gelido 15 mK sopra lo zero assoluto. Fonte: IBM
Il processore stesso è un piccolo chip incorporato in un circuito stampato largo solo pochi centimetri, che poggia sul fondo di un grande frigorifero di diluizione. Il chip è montato all'interno di uno schermo criopatico (una speciale schermatura magnetica che è più efficace a temperature criogeniche) al livello più freddo del frigorifero. Questa posizione è mantenuta a temperature inferiori a 15 milliKelvin - appena sopra lo zero assoluto e più freddo del vuoto dello spazio.
Il frigorifero di diluizione può raggiungere temperature così basse sfruttando il calore endotermico della miscelazione tra due isotopi di elio: elio-3 (3He) ed elio-4 (4He). Ciò è possibile perché la miscela 3He-4He subisce una separazione di fase spontanea al di sotto di 870 mK che si traduce in una fase concentrata di circa il 100% 3He e una fase diluita composta di circa il 6,6% 3He e 93,4% 4He. Quando le due fasi entrano nella camera di miscelazione sul fondo del frigorifero, si verifica un processo di diluizione quando 3 passa dalla fase concentrata alla fase diluita. Il calore viene estratto nel processo.
Questo aggeggio è necessario perché gli stati quantistici dei qubit nel processore sono estremamente delicati. Il minimo disturbo esterno - tra cui calore, vibrazioni e radiazioni elettromagnetiche - distruggerà gli stati quantici in un processo noto come decoerenza.
Nel frattempo, le microonde che trasportano i segnali di controllo e misurazione al chip quantico vengono trasmesse attraverso i cavi coassiali. Quando gli impulsi - oscillando a frequenze comprese tra 4 GHz e 6 GHz - passano attraverso ciascuno degli stadi del frigorifero, passano attraverso gli attenuatori per garantire che il rumore termico non raggiunga il processore.
Processore quantico
I chip quantici di IBM consistono in un wafer di silicio che contiene qubit e risonatori composti da alluminio e niobio. I qubit sono fatti in un modello generato con litografia a fascio di elettroni. Un risonatore è collegato a ciascun qubit per fornire gli impulsi di controllo che governano lo stato del qubit. I qubit sono prodotti mediante incisione di ioni di una pellicola di niobio spruzzato. Risonatori aggiuntivi collegano più qubit per abilitare l'entanglement.
Lo stato di un qubit entangled non può essere descritto indipendentemente dal suo qubit abbinato. Se viene misurato lo stato del primo qubit, il risultato della misurazione dello stato del secondo qubit è correlato a quello del primo. Utili computazioni quantistiche spesso implicano l'esecuzione di operazioni logiche condizionali in cui lo stato di un qubit dipende da quello di un altro qubit.
L'entanglement è uno dei fenomeni chiave della meccanica quantistica che fornisce il potenziale per un'immensa accelerazione computazionale tra computer quantistici rispetto ai computer classici. Un'altra chiave è la sovrapposizione, che consente ai qubit di esistere in più stati contemporaneamente. Il valore di un qubit è espresso come | 0⟩ o | 1⟩, analogo al classico bit 0 o 1. La differenza è che invece di esistere come solo 0 o 1, i qubit possono esistere in una sovrapposizione dei due valori.
Questa sovrapposizione può essere rappresentata geometricamente come una sfera di Bloch. Il polo nord della sfera rappresenta lo stato | 0⟩, mentre il polo sud rappresenta lo stato | 1⟩. Una sovrapposizione dei due stati è rappresentata da un vettore che punta dal centro della sfera a qualche punto sulla superficie tra i due poli.
Solo misurando lo stato del qubit il suo valore collassa a 0 o 1. La soluzione è come una distribuzione di probabilità: il qubit leggerà come 0 una certa percentuale del tempo e come 1 una percentuale diversa del tempo.
Questo comportamento è rappresentato dalla formula | ψ⟩ = α | 0⟩ + β | 1⟩ con | α | 2 + | β | 2 = 1. Quando misurata, la probabilità che il qubit crolli a 0 è | α | 2e il la probabilità che il qubit crolli a 1 è | β | 2. La possibilità di esistere in stati entangled e in sovrapposizioni di stati consente a qubits di valutare più soluzioni simultaneamente.
I processori quantistici di IBM utilizzano qubit transson basati su giunzioni a giunzioni fisse, superconduttori. Un qubit di questo tipo è essenzialmente un risonatore LC: un condensatore e un induttore collegati in serie. L'induttore è la giunzione Josephson - una coppia di elettrodi superconduttori che sono separati da un isolante molto sottile che è un millesimo di un capello spesso. La giunzione Josephson crea la non linearità che consente al risonatore LC di agire come un atomo con due livelli di energia quantistica: | 0⟩ e | 1⟩. Gli impulsi di controllo a microonde manipolano il qubit tra questi due stati per eseguire operazioni quantistiche.
Per determinare il risultato dell'operazione di un qubit, un segnale di misurazione viene inviato come impulso a microonde al risuonatore del qubit. La misura distrugge la sovrapposizione del qubit e collassa lo stato del qubit a 0 o 1. Il segnale riflesso viaggia sul frigorifero di diluizione, passando attraverso isolatori che proteggono i qubit dal rumore e amplificatori che aumentano la forza del segnale abbastanza da leggere lo stato del qubit con precisione. Il segnale quindi passa attraverso la pila di elettronica a microonde, questa volta per essere convertito dalla frequenza delle microonde a un segnale digitale che viene inviato su Internet al computer locale per visualizzare il risultato del calcolo quantistico.
Da:
https://electronics360.globalspec.com/article/13553/how-quantum-computers-work?id=-1474234620&uh=f9d092&email=giuseppecotellessa%40virgilio%2Eit&md=190326&mh=a59515&Vol=Vol19Issue17&Pub=1&LinkId=1978070&keyword=link_1978070&itemid=340933&et_rid=2064494091&et_mid=83839603&frmtrk=newsletter&cid=nl
Commenti
Posta un commento